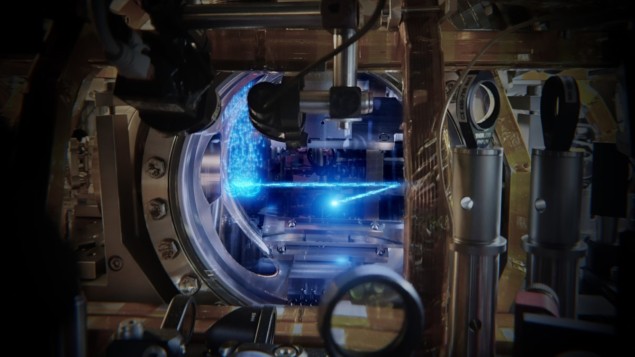
A continuous Bose–Einstein condensate (BEC) has been produced by researchers in the Netherlands. Claimed as a first, the achievement has been sought for years and could lead to continuous-wave atom lasers and a more fundamental understanding of the physics of condensed matter.
BECs form when a gas of bosonic atoms is cooled to ultracold temperatures. A large fraction of the atoms occupy the ground state of the system and the BEC behaves as a macroscopic system described by a single quantum wavefunction. BECs were first made in 1995 and their creators were rewarded with the 2001 Nobel Prize for Physics.
BECs are, strictly speaking, atom lasers, as quantum physicist Florian Schreck of the University of Amsterdam explains: “If you take the word laser as meaning light amplification by stimulated emission of radiation, and you translate all these words one by one to their atomic equivalents, then the process of making this macroscopically occupied mode is the same thing.”
A conventional laser beam is produced by drawing some of light out of an optical mode that exists within an optical cavity. To produce a continuous wave laser, one must pump energy into the cavity mode as fast as energy leaves via the laser beam and other loss processes.
Topping up atoms
One of the central goals of atom optics is to produce a continuous-wave atom laser – a system that delivers a continuous beam of coherent atoms. To achieve this, researchers would have to add new atoms to a BEC at least as fast as atoms in the beam left it.
Whereas photons are essentially non-interacting, ultracold atoms rapidly form molecules, which is usually the biggest cause of atomic loss in a BEC. To sustain a BEC continuously, therefore, physicists need to replenish these atoms rapidly and continuously. This alone has hitherto proved impossible, even without removing atoms to form a laser beam.
In 2013, Schreck and colleagues, then at Austria’s Institute for Quantum Optics and Quantum Information and the University if Innsbruck, created the first BEC by the laser cooling of atoms rather than evaporative cooling. Laser cooling is much faster and does not require most of the sample to be lost. They locked a laser to a narrow-linewidth atomic transition in strontium to cool a cloud of the atoms, while a second laser increased the trapping potential at the centre of the trap. This second laser made the centre transparent to the laser and allowed energy from these atoms, which heated up as their density increased, to diffuse away to the surrounding atoms.
Two-stage process
Laser cooling strontium atoms is a two-stage process, however: first the atoms are cooled from oven temperature using a broad, blue resonance, then a second, much narrower resonance cools the atoms from 1 mK to around 1 μK.
“Unfortunately, this trick that we used to protect the BEC from the laser cooling photons doesn’t work for the broad linewidth laser,” explains Schreck; “So we first cooled our sample using the blue light, then we switched that off.” This sequential approach could only produce a condensate intermittently, therefore.
In the new work, Schreck and colleagues designed a new machine with two separate vacuum chambers. This allowed them to guide a beam of atoms through both and replenish the BEC continuously.
“Instead of executing all these cooling transitions one after the other in time as people always did before, you’re executing them one after the other in space,” explains Schreck. The result was a condensate that was replaced faster than it decayed, allowing it to persist indefinitely.
Great progress
Several groups have previously attempted to implement sequential cooling stages using multiple techniques to cool a variety of atoms, Shreck says. “They made great progress, but they weren’t able to push it all the way through. Now the technology is just more mature, and strontium is just nice because it has this narrow linewidth cooling transition, which made it easier for us.”
The researchers’ main goal is continuous-wave atom lasers, which could find a host of uses in gravitational wave detection, dark energy searches, tests of the equivalence principle and elsewhere. Schreck says it is unclear precisely how much of the beam could be extracted at present as simulations are imprecise, but he is “absolutely confident it is more than 20%” and believes increasing the gain further should prove relatively simple.
Continuous atom laser one step closer, say physicists
Beyond atom laser beams, however, continuous BECs could answer important questions in condensed matter physics. “This is a driven, dissipative system and you can, have, in principle, novel quantum phases and quantum behaviour in dynamic systems where there’s drive and dissipation,” says Schreck, adding. “Theorists are quite interested in this”.
Jun Ye of JILA at the US National Institute for Standards and Technology and the University of Colorado is impressed. “Florian Schreck’s group has been working on a continuous source of ultracold strontium atoms for a number of years,” he says. “It is really satisfying to see that they have made a major breakthrough in combining this technology with a continuous Bose-stimulated scattering of thermal atoms into a Bose-Einstein condensate of strontium-84. This technology, once extended with a capability of continuous output, will have big impact for quantum sensors ranging from matter-wave interferometers to clocks.”
The research is described in Nature.