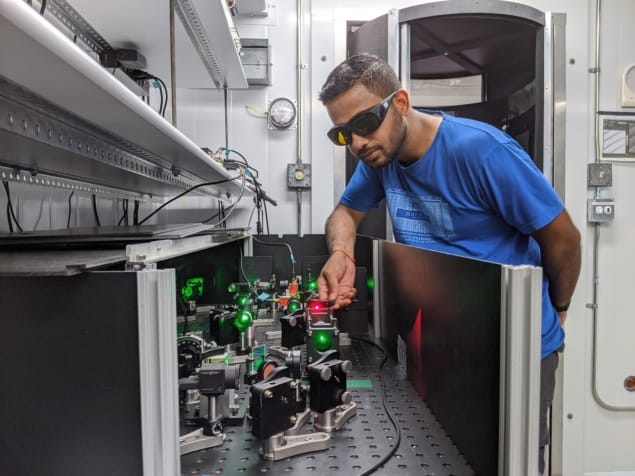
In 1963, a Tanzanian schoolboy called Erasto Mpemba was making ice cream when he noticed something strange: hot water sometimes freezes faster than cold water. Though Mpemba was not the first to wonder about this phenomenon, his report nevertheless captured the scientific imagination. The so-called “Mpemba effect” has remained contentious ever since – not least because the complex matrix of interactions at work when freezing a cup of hot water, coupled with water’s many anomalies, make it difficult to reproduce.
Researchers at Simon Fraser University in Canada have now overcome this problem with a simplified experimental model of a hot system relaxing to equilibrium with a colder heat bath. According to team leader John Bechhoefer, the technique he developed with PhD student Avinash Kumar enabled them to replicate the Mpemba effect in a reliable way, making it possible to pin down the precise conditions it requires.
A simplified model system
The definition of the Mpemba effect has been hotly debated. Is it concerned with the time it takes for water in a container to start to freeze, completely freeze (both of which are hard to observe accurately in practice) or simply to reach freezing temperatures? To complicate matters further, a similar effect has also been observed in magnetic systems, for other phase transitions. However, these systems, although simpler than water, are still too complex to pin down precise parameters for the effect.
Bechhoefer and Kumar’s experiments start with a beaker of water, but they don’t change the water’s temperature in real terms. Instead, they release a tiny glass bead in the beaker thousands of times from different positions across the width of a sample that defines the effective container size for their experiment. The locations where they release the bead are determined according to a probability distribution defined by thermodynamics and Boltzmann statistics for the system’s chosen initial “temperature”.
As the bead falls it is bombarded by water molecules, resulting in Brownian motion, but the researchers also subject it to a “virtual potential profile” using a feedback optical tweezer system. This changes the probability distribution of the bead’s position – effectively creating a change in the system’s “temperature”. This virtual potential profile has two dips in it, in line with the double potential well of water’s free energy landscape: one dip where water can “supercool” to liquid water at subzero temperatures, and a lower dip where water freezes. By measuring the probability distribution of the released beads’ positions after a set time, and establishing how much that distribution differs from an equilibrium probability distribution, the researchers gain information about how quickly the system is equilibrating – an analogue to how quickly a cup of water would approach freezing temperatures.
While all this may sound more complicated than popping a cup of water in an ice box, Bechoefer says it actually makes it a lot simpler to determine what conditions are required to produce the Mpemba effect. The idea arose out of a visit to the University of Maryland, where Zhiyue Lu and Oren Raz were then working on a theoretical framework for a simplified model of the Mpemba effect. During the visit, Lu and Raz button-holed Bechhoefer and persuaded him that his specialist feedback optical tweezer system – which allows finer, non-diffraction-limited control over the virtual potential landscape than regular optical tweezers – would make a great way of testing their model. Thanks to this system, Bechhoefer and Kumar could work with a sample that had an effective width of just 0.4 µm, making experimental runs much faster.
Reliably reproducing the Mpemba effect
Bechhoefer acknowledges that his team’s system is an “abstract” and “almost geometrical” way of picturing the Mpemba effect. Nevertheless, he and Kumar were able to identify parameters for which hotter “initial temperatures” cooled faster than chillier ones. “It sort of suggested that all the peculiarities of water and ice – all the things that made the original effect so hard to study – might be in a way peripheral,” he says.
Although the double potential well played a crucial role in producing the effect, Bechhoefer and Kumar found this alone was not enough to trigger it. The system also needed the barrier between the two potential wells to be offset from the midpoint between them. When the distance to the deeper well is greater than the distance to the shallower well, the researchers found that the number of starting positions for which the bead will fall directly into the deeper well (rather than entering the shallower well first and then jiggling around until Brownian motion eventually nudges it into the deeper one) is greater.
While the Mpemba effect is not typical behaviour, Bechhoefer and Kumar’s study suggests that it is not limited to specialized conditions that would make it impossible to reproduce in a reliable way. “What this shows is that there are systems where you can reproducibly not just observe, but in some sense create, engineer and control the effect,” Bechhoefer says.
Controlling the Mpemba effect could have important practical implications too, for example in the heat removal systems that keep electronics cool. Lu and Raz’s theoretical treatment also suggests that there should be a “reverse Mpemba effect” for heating systems, and Bechhoefer and Kumar have their sights set on replicating this in future experiments.
When cold warms faster than hot
“This work extends the Mpemba phenomenon,” says Changqing Sun, whose research at Singapore’s Nanyang Technological University focuses on properties of water that relate to the Mpemba effect. However, while Sun asserts that the work “certainly contributes to the knowledge for general situations of heat removal” he thinks further caution may be needed when applying it to water. In water, he adds, “the microscopic mechanism may differ, such as the higher thermal diffusivity and lower specific heat of water surface and the manner of hydrogen bond (O:H-O) energy exchange.”
Full details are reported in Nature.