Cause-and-effect explanations like “catnip causes cats to be happy”, “jokes cause laughter” and “exciting research causes Physics World articles” are a useful way to organize knowledge about the world. The mathematics of cause-and-effect underpin everything from epidemiology to quantum physics. In the quantum world, however, the link between cause and effect is not so straightforward. An international team of physicists has now used quantum violations of classical causality to better understand the nature of cause-and-effect. In the process, the team uncovered quantum behaviour in a situation where standard methods indicate that the system ought to be classical – a result that could have applications in quantum cryptography.
In quantum physics, a result known as Bell’s theorem states that no theory that incorporates local “hidden” variables can ever reproduce the correlations between measurement outcomes that quantum mechanics predicts. A similar result occurs in the theory of causal inference, where quantum systems likewise defy the rules of classical causal reasoning. The idea behind the causal inference approach is that while a statistical correlation between two variables can arise due to a direct causal relationship between them, the correlation may also contain the contribution of a hidden common cause. In some cases, this hidden contribution can be quantified, and this can be used to show that quantum correlations do exist even when Bell’s theorem cannot be violated.
Inferring causal structure achieves direct control over cause and effect
In the latest work, a team led by experimental physicist Davide Poderini and colleagues in Brazil, Germany, Italy and Poland combine theory and experiment to show quantum phenomena in a system that would otherwise appear classical. The researchers explore the notion of cause and effect by considering whether correlations between two variables, A and B, imply that one is the cause of the other, or whether some other (potentially unobserved) variable may be the source of the correlations.
In their investigation, the researchers use a causal model (see image) in which the statistics of variable A influence those of variable B, either directly or by the action of a common source (called Λ) that connects the outcome of both variables even without the presence of a causal link between them. To distinguish between these two scenarios, the researchers perform an intervention on variable A that erases any external influences. This leaves the variable A under the experimenter’s complete control, making it possible to estimate the direct causal link between A and B.
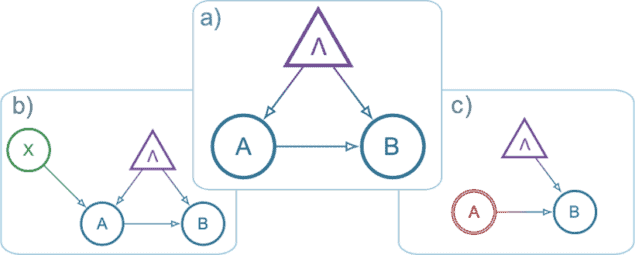
Alternatively, by introducing an additional variable X that is independent of B and Λ, any observed correlations between variables A and B can be decomposed into conditional probabilities. These conditional probabilities place a lower bound on the degree of causal effect between the variables, making it possible to estimate the level of influence between A and B.
The researchers call this lower bound an instrumental inequality, and it is a classical constraint that (similar to the inequality that arises from Bell’s theorem) stems from imposing this causal structure on an experiment. As a result, the degree of quantum causal influence between variables A and B will be less than the minimum required for a classical system, allowing nonclassicality to be observed through an intervention even when no Bell inequality is violated.
Experimental intervention reveals quantum effects
To observe the instrumental causal process, the researchers generated pairs of photons with entangled polarizations and measured them in different representations of state space, or bases. Thanks to photons’ entangled nature, the choice of basis for one is determined by the measurement on the other, producing a “feed-forward” mechanism that implements a direct causal link between the two variables. As a result of this feed-forward process, the researchers experimentally observe violations of the classical lower bounds for causal influence between two variables by producing several quantum states characterized by different degrees of entanglement.
Quantum mechanics trumps nonlocal causality
Like Bell’s inequality, violation of this classical lower bound represents a signature of quantum correlations. Furthermore, it yields statistical data that can act as the foundation of any basic quantum cryptographic protocol. While current cryptographic protocols rely on Bell’s theorem, inferring causal structure from instrumental intervention represents a more general compatibility between classical causality and quantum theory. Poderini and his colleagues seek to experiment with different causal scenarios to explore complex networks with richer correlations, which can be exploited to develop novel quantum technologies. The researchers believe their experimental techniques could lead to quantum advantages in cryptographic protocols, making it possible to realize more resilient and less technologically demanding cryptographic tools.