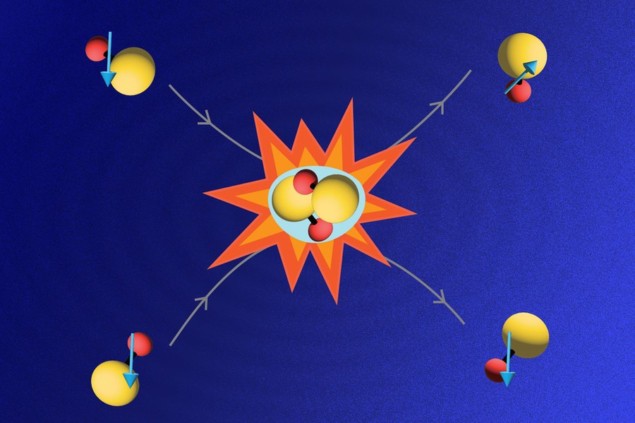
A way of colliding ultracold molecules while controlling the rate at which they react has been developed by physicists at the Massachusetts Institute of Technology (MIT) in the US. Researchers at Germany’s Max Planck Institute for Quantum Optics have made a similar discovery using an different experimental technique. Their research opens new pathways for enhanced control of chemical reactions.
Chemical reactions are immensely complex, with huge numbers of atoms and molecules colliding with each other while being driven by kinetic forces. This complexity makes it very difficult to focus on reactions at the atomic and molecular level.
To get around this complexity problem, researchers can cool atoms and molecules to microkelvin temperatures to limit the possible quantum states the reactants can be in. Reactions involving these ultracold atoms and molecules can then be partially controlled using lasers or magnetic fields, providing important information about chemical processes.
One challenge in studying ultracold molecules is that they have rotational and vibrational quantum states. This makes molecules much more difficult to control than atoms, and this has prevented ultracold experiments from moving beyond simple atom–atom and atom–molecule reactions.
Feshbach resonances
Now, a team at MIT led by the Nobel laureate Wolfgang Ketterle has developed a new way of controlling of ultracold molecules. The technique uses Feshbach resonances, which occur when two colliding atoms or molecules briefly form a bound state. Feshbach resonances are widely used in the study of ultracold gases because they can be used to fine tune interactions between atoms.
Applying Feshbach resonances to ultracold atoms was pioneered by Ketterle in 1998, when he made the first ever observation the phenomenon in ultracold sodium atoms. Since then, researchers have been searching for similar resonances in collisions involving both atoms and molecules. Last year, Ketterle and colleagues used Feshbach resonances to create reactions involving sodium atoms and sodium-lithium molecules. They found that quantum interference effects related to multiple bounces between colliding particles can be constructive or destructive. This either enhances or suppresses the reactions by factors of about 100.
Now the MIT researchers have found a Feshbach resonance in collisions between pairs of ultracold sodium-lithium molecules. It occurs within a very narrow range of the applied magnetic field. When the researchers looked over a magnetic-field range of more than 1000 G, they found an increased reaction rate between molecules in a narrow 25 mG window. The team concluded that the Feshbach resonance encouraged the molecules to move into a relatively long-lived intermediate complex that in turn increased the number of molecular reactions up to 100 times.
Big surprise
Further analysis of the new data yielded a surprising discovery. Precisely at the resonance, two states of the molecule have exactly the same energy and therefore can both take part in the collision. Even though the result was unexpected, Ketterle points out that sodium–lithium is the lightest ultracold molecule being studied. As a result, it has the smallest density of states and that therefore it is highly likely that the molecule has an isolated state that is long-lived.
To understand their observations, the team developed a model that describes the resonance caused by the magnetic field and the decay of the intermediate complex into an open channel causing the molecule to disappear.
Their model is analogous to light resonating within a Fabry-Perot cavity – a device comprising two thin mirrors that will transmit light at a specific resonant wavelength. The lifetime of the intermediate complex is analogous to round-trip time that a photon spends inside a resonant cavity.
While this model explains the results, some open questions remain. For example it would be useful to know if these narrow resonances are unique to molecules with small atoms – molecules that have a lower density of states. It would also be of interest to explore whether other magnetic-field values create long-lived complexes. Undoubtedly these questions will spark a wave to excitement in the field of ultracold chemistry and could lead to new applications and physical insights.
In control
Ketterle believes that the research will prove to be important for quantum science, physical chemistry and chemistry. But he acknowledges that more work needs to be done and that without a full understanding of the resonance it is difficult to make predictions for other molecules. However, he says that his team’s observation has made it more likely that resonances and long-lived collision complexes exist in other molecules.
Physicists close in on a simpler route to quantum degenerate molecules
“The field is currently progressing towards control at the quantum level over more and more complex systems. Our work is a step to achieve quantum control over molecular collisions and reactions and to map out more broadly the collisional properties of these molecules with the goal of finding a deeper understanding”, he tells Physics World.
Bo Zhao from the University of Science and Technology of China lauds the team’s discovery of a magnetically tunable Feshbach resonance between ultracold ground-state diatomic molecules, adding that the work is an important advance in ultracold molecules and ultracold chemistry. He states that Feshbach resonances between molecules could lead to many new research possibilities, including the study of strongly interacting molecular gases.
The research is described in Nature. In the same issue of the journal, Xin-Yu Luo and colleagues at Germany’s Max Planck Institute for Quantum Optics describe a similar scheme for controlling the reaction rate of ultracold sodium–potassium articles. In this research, the team used oscillating microwave radiation to create the resonance.