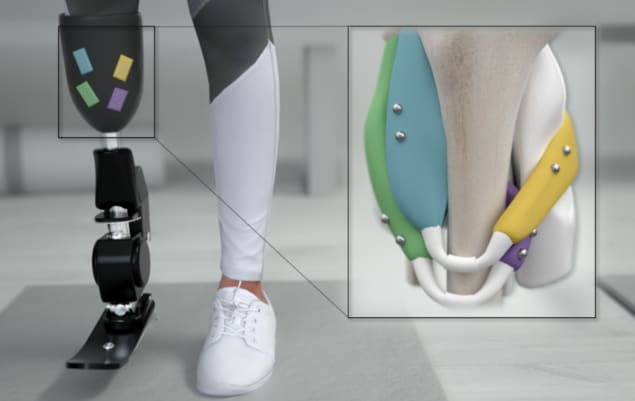
In recent years, health and fitness wearables have gained popularity as platforms to wirelessly track daily physical activities, by counting steps, for example, or recording heartbeats directly from the wrist. To achieve this, inertial sensors in contact with the skin capture the relevant motion and physiological signals originating from the body.
As wearable technology evolves, researchers strive to understand not just how to track the body’s dynamic signals, but also how to simulate them to control artificial limbs. This new level of motion control requires detailed understanding of what is happening beneath the skin, specifically, motion of the muscles.
Skeletal muscles are responsible for almost all movement of the human body. When muscle fibres contract, the exerted forces travel through the tendons, pull the bones and ultimately produce motion. To track and use these muscle contractions in real-time and with high signal quality, engineers at Massachusetts Institute of Technology (MIT) employed low-frequency magnetic fields – which pass undisturbed through body tissues – to provide accurate and real-time transcutaneous sensing of muscle motion. They describe their technique in Science Robotics.
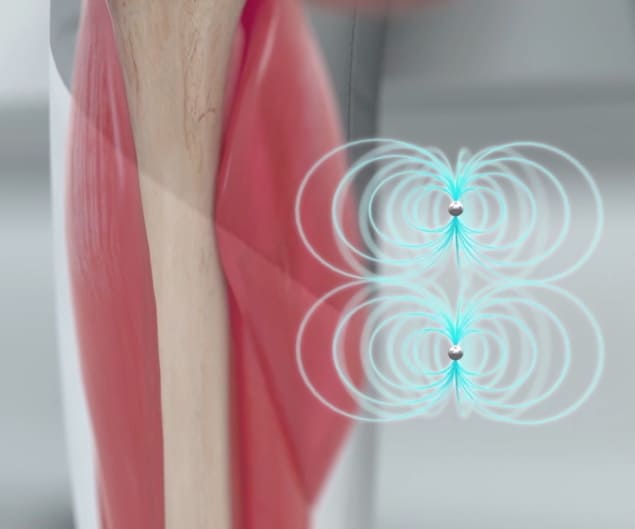
Engineers at the MIT Media Lab implanted pairs of tiny magnetic beads into the leg muscles of four turkeys and applied a mechanical frequency sweep to create leg motion. An external array of magnetic field sensors tracked the spatiotemporal changes in muscle length when the turkey moved.
This real-time magnetic tracking strategy, called magnetomicrometry, demonstrated a submillimetre tracking precision of around 37 µm – comparable to the current gold-standard method for tissue position measurements.
“We are currently studying the use of magnetomicrometry in a mobile context to verify that motion of the sensor array (relative to the muscle frame as well as to the world frame) does not cause any unexpected issues with the tracking,” explains lead author Cameron Taylor, a postdoctoral associate at MIT Media Lab.
Inside the body – biocompatible magnets
Using a minimally invasive percutaneous injection, the team implanted pairs of 3-mm diameter magnetic beads at different locations within the animals’ leg muscles. The bead pairs were strategically placed at least 21.5 mm apart to prevent bead migration caused by pulling forces when two magnets are in close proximity.
This cut-off distance can be determined empirically for a given bead size and magnetization strength to reduce the migration risk and increase safety.
Before implantation, all magnets were coated with a biocompatible and corrosion-resistant polymer to mitigate the risk of infection or foreign body reactions. Twenty-seven weeks after implantation, harvested tissue revealed no adverse reactions to the presence of the magnetic beads.
Outside the body – tracking array
To monitor muscle movements, the team mounted a portable 96-sensor array externally on the turkeys’ legs. The array captures spatiotemporal changes in the surrounding magnetic field caused by changes in the relative distances between magnetic beads during motion. A time derivative of the magnetic bead distance yields the velocity, and a linear transformation gives the local strain in the muscle, both key control parameters during prosthetic manipulation.
The tracking accuracy is highly influenced by the number of sensors, the subcutaneous sensing depths and the strength of each magnet. The researchers note, therefore, that a balance between sensor size, which impacts portability, and accuracy must be maintained for successful wireless sensing.
Combining magnetomicrometry with exoskeletal control can potentially impact many people undergoing physical therapy and rehabilitation. “A person with injured or weak muscles, for instance, could have pairs of magnets implanted in those muscles for the control of an exoskeleton that restores or augments their strength,” Taylor tells Physics World.
