
In any normal year, the American Association for Physicists in Medicine (AAPM) would be preparing to welcome around 4000 attendees to its annual meeting and exhibit, which in 2020 was due to take place in Vancouver, Canada, as a joint meeting with the Canadian Organization for Medical Physicists (COMP). But this year will be different: the bustle of the traditional conference venue will be replaced by a virtual meeting platform that will allow delegates to attend scientific presentations, take part in live Q&A sessions, and interact with leading equipment vendors in an online exhibit hall.
The scientific programme for the Joint AAPM | COMP Virtual Meeting will feature six tracks of specially curated presentations, along with a series of interactive online ePosters. Delegates can opt to attend the live presentations, which will each be followed by an interactive Q&A, or catch up a via an on-demand service that will be available for six weeks after the event.
The virtual meeting platform will also host networking and social events, such as an online fitness challenge that will enable delegates to earn points for completing a daily 30-minute activity. Meanwhile, the online exhibit hall will be open for delegates to browse company and product information, view video presentations, and schedule meetings with almost 60 exhibitors. A vendor-focused seventh track of presentations offers delegates a deeper dive into specific products and services, with experts available to answer questions immediately after their talks. As a taster, some of the latest innovations from the vendor community are highlighted below.
Robotic radiosurgery system delivers precision, speed and motion synchronization
The CyberKnife S7 System from Accuray is the first robotic and fully automatic system for stereotactic radiosurgery (SRS) and stereotactic body radiation therapy (SBRT), enabling clinicians to deliver personalized treatments to more patients. The system delivers non-surgical stereotactic treatments in any part of the body with sub-millimetre precision, with powerful motion tracking capabilities and adaptive treatment planning to enable high doses of radiation to be targeted directly at the tumour.
The CyberKnife system offers real-time motion synchronization to automatically adapt the treatment in response to any movement of the target or patient. It exploits Accuray’s proven Synchrony technology, which uses artificial intelligence to continuously synchronize the radiation beam to the target location.
Such real-time adaptation allows clinicians to escalate the radiation dose delivered to the tumour while minimizing the exposure of healthy tissue, and also enables more precise and effective hypofractionation treatments. The fully automated system ensures there is no impact on standard workflows or delivery speed, while also avoiding unnecessary manual tasks and reducing the potential for error.
The CyberKnife S7 is the first SRS/SBRT system to have a linear accelerator mounted on a robotic arm, which provides flexibility and freedom of motion for delivering complex beam profiles to any part of the body. An integrated stereoscopic X-ray system provides continual image guidance and real-time tracking information to maintain accuracy and precision during treatment.
The fully automated system is designed for operational efficiency, providing personalized treatment planning while also maintaining patient throughput. Treatment plans can be optimized in as little as 60 seconds, in some cases enabling same-day planning and treatment delivery, while hypofractionated treatments can be delivered in just 15 minutes.
More information about the CyberKnife S7 System can be found on the Accuray website.
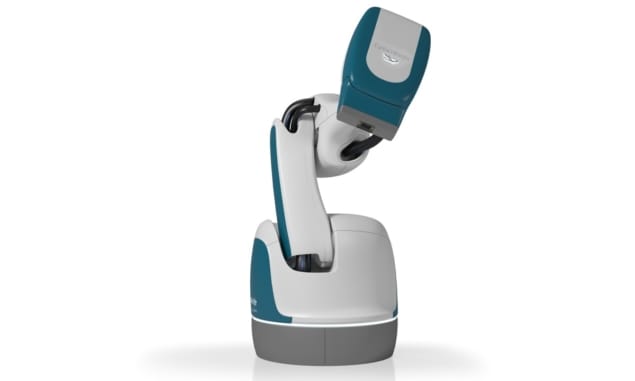
Seeing is believing: a new way to visualize radiotherapy
The BeamSite video imaging system from start-up company DoseOptics enables clinicians to visualize in real time how the radiation beam is being delivered to the patient. The system captures video-rate images of both the entry and exit beams during treatment, and also provides record and playback capabilities for sharing observations and investigating any anomalies with the clinical team.
The BeamSite system offers direct video imaging for most common radiotherapy modalities, as well as total skin electron therapy. Such real-time visualization is particularly useful for monitoring any stray radiation during treatment, checking that the patient remains in the correct position while the beam is being delivered, and identifying and understanding any errors or near misses.
The system works by detecting the faint Cherenkov radiation that is emitted by human tissue when it is irradiated with high-energy electrons or photons. Unique time-gating technology ensures that each pulse of radiation delivered by the linac contributes to the image recovered, and time-integrating software accumulates the Cherenkov emission to create an image that overlaid in real time on the area being irradiated. The camera and software operate remotely to provide an independent check and measurement tool for beam shape and delivery.
The team from DoseOptics will be presenting several talks and posters during the virtual AAPM meeting to explain the BeamSite system in more detail. They will be:
Talk: Treatment Verification from Cherenkov Imaging During Radiation Therapy Sunday 12 July, 14.00 ET
Talk: First Imaging of Intrinsic Light Emission from Biological Tissue Visualized Proton Pencil Beam Scanning Monday 13 July, 15.30 ET
ePoster: Evaluating the Clinical Utility of Cherenkov Imaging in Radiotherapy
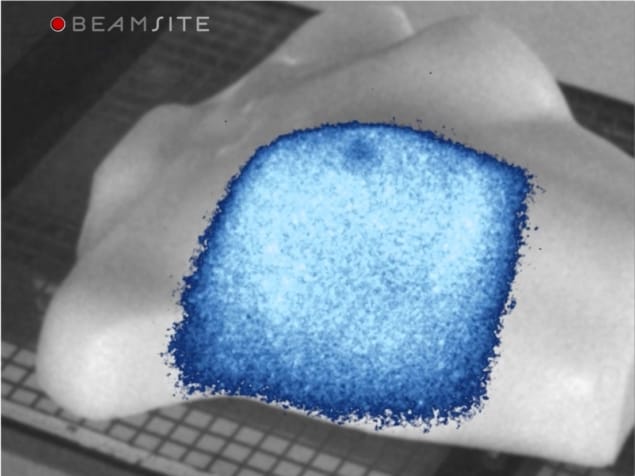
Optimized DCAT planning delivers faster treatments
A recent study by medical physicists at the UT Health San Antonio Cancer Center reveals that patients with simple lung or liver lesions could be treated more efficiently – and just as effectively – with optimized dynamic conformal arc therapy (DCAT). While traditional DCAT generally does not achieve the same plan quality as volumetric modulated arc therapy (VMAT), optimizing the DCAT delivery by varying the dose rate and gantry speed delivers highly conformal dose distributions.
“At our institution, this would mean that 40–60% of lung and liver patients who would normally be treated using VMAT could be treated more efficiently using optimized DCAT, while maintaining the same plan quality,” conclude Sotiri Stathakis and Niko Papanikolaou. “These patients could benefit from significantly shorter treatment times, which are easier to tolerate and reduce the risk of intrafraction movement.”
Stathakis and Papanikolaou exploited the Monaco treatment planning system from Elekta, which offers a variable dose-rate feature for DCAT delivery. It also includes segment shape optimization for DCAT, which optimizes the beam weights and shapes to improve conformality and prevent damage to healthy organs.
Comparing the plans produced for 19 patients using both VMAT and optimized DCAT revealed that both techniques achieved the same plan quality when the targets were located away from other critical organs. But DCAT could be delivered 2.5 times faster than VMAT – which could be particularly beneficial for treatments exploiting the deep-inspiration breath-hold technique.
“Based on the results of this study, lung and liver patients with simple, spherical lesions that are not close to organs-at-risk are ideal candidates for optimized DCAT,” conclude Stathakis and Papanikolaou. “The potential for application of optimized DCAT to other treatment sites, such as pancreas, brain and prostate, is of great interest and the subject for future investigation.”
Read more about the study in a white paper available from Elekta.
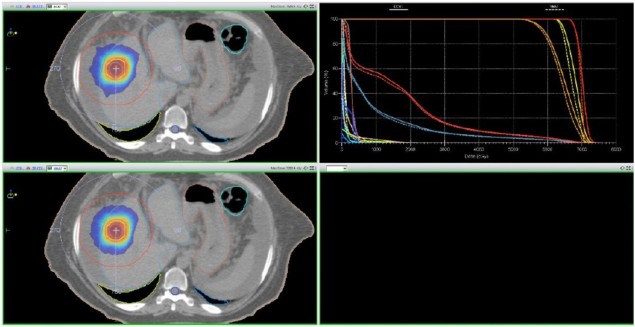
Adaptive radiotherapy delivers intelligent treatments
The new Ethos™ therapy system from Varian enables clinicians to adapt radiotherapy treatments to daily changes in the patient’s anatomy. It has been designed to help deliver treatments that better target the tumour, reduce the dose to healthy tissue, and achieve the clinical objectives for each treatment plan.
Ethos incorporates artificial intelligence (AI) to increase the capability, flexibility and efficiency of radiotherapy. It has been designed to allow physicians to assess and adapt treatment plans daily, enabling them to deliver more personalized cancer care and offering the potential for improved patient outcomes.
Ethos therapy integrates Iterative cone-beam CT and multimodality images on the treatment console, providing clinicians with an up-to-date view of the patient’s anatomy to help make better informed treatment decisions. The streamlined workflow of Ethos therapy is enabled by its AI-driven planning and contouring capabilities, making it possible to better visualize daily changes and enabling physicians to make any adjustments to the treatment within minutes.
Find out more by visiting varian.com/ethos and downloading the Ethos therapy brochure.

Optimization technique enables more effective treatment planning
RaySearch Laboratories explains in a new white paper how multi-criteria optimization (MCO) helps clinicians select the optimal treatment plan for their patients through a streamlined and intuitive workflow. The company’s RayStation treatment planning system supports MCO for intensity-modulated radiotherapy, as well as volumetric modulated arc therapy, tomotherapy and proton pencil-beam scanning.
Such MCO techniques start with a series of ideal clinical objectives, such as delivering a uniform dose to the target volume and zero dose to critical organs, from which a series of possible plans are generated. Clinicians can explore these different plans through RayStation’s interactive interface, which exploits slider controls to alter the dose distribution and examine in real time the impact on the defined clinical goals.
As well as such manual navigation, the module also offers an automatic option that optimizes the treatment plan based on a prioritized list of clinical objectives. The dose distribution selected through the chosen navigation process can be converted to machine parameters through a dose mimicking optimization that minimizes discrepancies between the navigated dose and the deliverable plan.
A number of research studies have shown how MCO can speed up the treatment planning process, while also delivering higher quality plans than standard inverse planning. MCO has also been shown to enable novice dosimetrists to create treatment plans of comparable quality to more experienced planners using traditional techniques, while a proof-of-concept suggests that MCO could enable treatment plans to be created in a single meeting between a physician and a planner to save time and improve clinical decision making.
Read the full white paper on the RaySearch website.
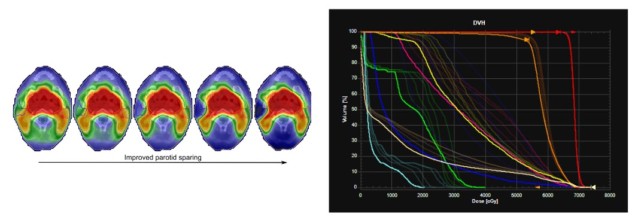
Autocontouring delivers equivalent results in half the time
DLCExpert is an automatic contouring system from Mirada Medical that exploits artificial intelligence and deep learning to delineate organs-at-risk and other anatomical structures, which is an important but time-consuming element of radiotherapy treatment planning. An expert assessment by oncologists at MAASTRO Clinic in the Netherlands suggests that the time needed for thoracic contouring can be reduced from 20 minutes when done manually using existing clinical routine to just 10 minutes with the deep-learning approach, while validation results available from Mirada show that DLCExpert produces OAR contours of a similar quality to those drawn by professional clinicians.
DLCExpert exploits Mirada’s Zero-Click Contouring platform, which uses background processing to deliver contours before planning gets underway. Contours can be validated using any treatment planning software, or the RTx imaging workstation available from Mirada.
DLCExpert supports all major anatomical sites including breast, lung, head and neck, and prostate. To test out its capabilities, visit www.autocontouring.com to take Mirada’s modified version of the Turing test to try to identify which contours are drawn by radiation oncology professionals and which have been automatically drawn by Mirada software.
More information about the deep-learning techniques used in DLCExpert can be found in a technical white paper from Mirada Medical.
Imaging guides proton therapy
Tony Lomax, Chief Medical Physicist at the Paul Scherrer Institute in Villingen, Switzerland, offers an insight into the different imaging modalities that are needed for proton therapy in a new white paper for Siemens Healthineers. Most important for making initial clinical decisions are the offline imaging techniques used to diagnose the disease, which determines whether the patient will be treated with protons or conventional radiotherapy, and to map out the extent of the tumour and any critical structures and organs nearby.
Such anatomical imaging is generally achieved with computed tomography and magnetic resonance imaging, which offer excellent spatial resolution and good anatomical contrast. MR imaging is also used to image the activity and function of the tumour, often in combination with positron-electron tomography (PET). Lomax points out that it can be difficult to match these two datasets, since PET has a lower spatial resolution and does not provide anatomical information, which means that imaging systems such as PET-CT and PET-MRI are now emerging to improve the precision with which the tumour can be defined.
For proton therapy it is particularly important to understand how the tumour or surrounding organs might move during treatment, which requires time-resolved images to be captured before treatment gets underway. By far the most popular is 4D CT, although major artifacts in the reconstructed data can be caused by any variability in the patient’s breathing. Interest is therefore growing in other techniques, such as 4D MRI, that can reduce these motion artifacts and also capture data over longer periods of time.
Lomax also reviews the options for online imaging during proton therapy. Commercial proton therapy machines are now equipped with cone-beam CT (CBCT) systems for in-room 3D imaging, which is widely used to ensure that the patient is positioned in the same way for each treatment. But some proton centres have opted instead for in-room CT systems that provide the same diagnostic quality as pre-treatment CT, and which also offer useful information for adapting the treatment in response to any anatomical changes, such as the size and shape of the tumour.
While MR-guided systems are now emerging for real-time imaging in radiotherapy, Lomax points out that integrating MR with a proton machine presents several practical challenges that have yet to be overcome. Online 4D imaging is also not yet available for proton therapy, which means that real-time motion tracking must instead rely on time-resolved 1D or 2D imaging. Lomax highlights two recent studies in which such 2D surrogates based on X-ray fluoroscopy or ultrasound monitoring have been used to reconstruct 3D movements using motion models derived from 4D MRI imaging.
Read the full white paper by Tony Lomax on the Siemens Healthineers website.
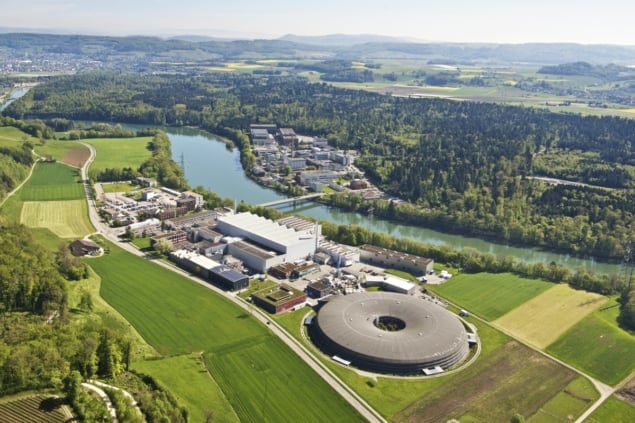