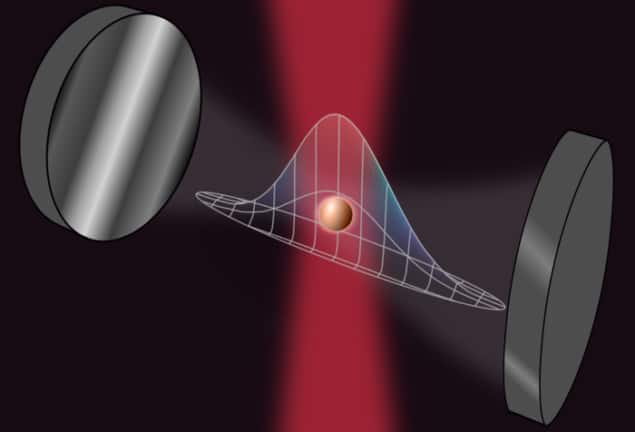
The motional fluctuations of nanoparticles trapped between mirrors in optical cavities could form the basis for a new type of high-precision quantum sensor, according to calculations by researchers at the Austrian Academy of Sciences, the University of Innsbruck in Austria and ETH Zurich in Switzerland. The team’s theoretical work shows that the dynamical instabilities of a quantum system can be exploited as a resource rather than being considered as a problem to be avoided, as is usually the case.
Optical cavities, or resonators, are structures in which light is reflected back and forth between two or more mirrors. This light interacts with nanoparticles trapped in the cavity, creating dynamical instabilities that are usually considered undesirable for sensing applications.
While optical cavities are routinely used to cool the motion of levitated particles via successive momentum “kicks” from reflected photons, these structures need to be relatively large to keep a photon inside the cavity for longer than the oscillation period of a nanoparticle. However, Romero-Isart explains that if the goal is not to cool the nanoparticles’ motion, but to squeeze it, the requirement is different. Here, much like a balloon, the nanoparticles are squeezed in one direction in position-momentum space, which creates a bulge in the other direction. This generates stronger light-nanoparticle coupling, which can be achieved using smaller cavities.
The researchers applied their theoretical approach to a model system consisting of levitated silica nanoparticles coupled to a microcavity. Squeezing the motion of particles is helpful, Romero-Isart says, because it makes them more sensitive to external signals – something that can be exploited for inertial and force sensing. Optical resonators therefore offer a new platform for the design of quantum sensors that could be used, for example, in satellite missions, self-driving cars and in seismology, to name but three examples.
Crystalline supermirrors cut optical losses
According to the researchers, the study is timely because other research groups have recently demonstrated ground-state cooling and quantum control of optically-levitated nanoparticles in free space in laboratory experiments. The team is now looking into how to prepare macroscopic quantum superpositions of a nanoparticle. “This means preparing it in a state in which its position is delocalized over length scales comparable to the size of the nanoparticle,” Romero-Isart tells Physics World.