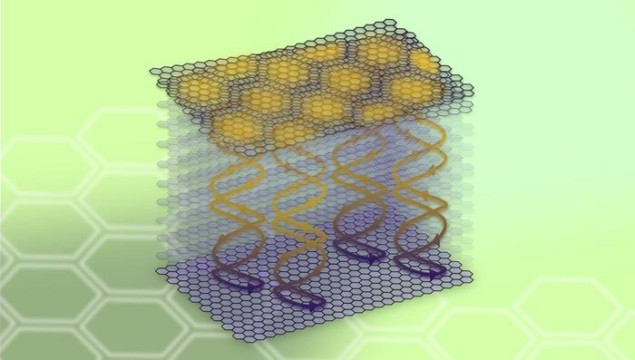
When two sheets of graphene are placed on top of each other and slightly twisted, their atoms form a moiré pattern, or superlattice. At the so-called “magic” twist angle of 1.08°, something unusual happens: the weak van der Waals (vdW) coupling between atoms in adjacent layers modifies the atoms’ electronic states and transforms the material from a semimetal to a superconductor. The study of such twist-related electronic effects is known as “twistronics”, and it also includes phenomena such as correlated insulator states that appear at different degrees of misalignment.
Because the moiré pattern that underlies twistronics appears only at the interface between two thin sheets, it was assumed that twistronic effects could only occur in structures containing just a few layers. Although it is possible to produce a moiré pattern at a two-dimensional interface within a three-dimensional structure, it was thought that this pattern would not substantially modify the properties of the bulk material. After all, the 2D moiré region would only comprise a small fraction of the total 3D crystal volume.
New work by two research groups – one at the University of Washington in the US and Osaka University in Japan, the other at the University of Manchester in the UK – shows that this picture is not always correct. In fact, rotating a single layer of a 2D material by a small twist angle within a three-dimensional graphite film can cause the properties of the moiré interface to become inextricably mixed with those of the graphite. The result is a new class of hybrid 2D-3D moiré materials that substantially alters our understanding of how twistronics works.
A profound and exciting transformation
The usual method of making vdW materials begins with cutting a single monolayer in half. The two halves are then stacked on top of each other with a finely-controlled twist angle between them.
The Washington-Osaka researchers used a similar procedure, but with a different starting point. “We started with exfoliated (peeled off) flakes of bulk graphite (that is, 10 or more layers) that had a small area of monolayer graphene attached,” explains team leader Matthew Yankowitz, a physicist at Washington. “We then cut the graphene to separate it from the bulk graphite and stacked the two portions with a twist angle between them.”
To observe the effects of the resulting moiré pattern, the researchers cooled the twisted graphene-graphite structure down to cryogenic temperatures and measured its resistance as they applied a magnetic field. Even when this field was small or zero, they found that the modified electronic states on the moiré surface produced different electrical transport properties than those found in standard graphite.
“We can see this by doping charges into each surface using nearby gate electrodes,” Yankowitz says. Because graphite is a semimetal, it contains fewer charge carriers (electrons and holes) than a normal metal. Adding a substantial amount of charge to the moiré surface therefore gives this surface an outsize influence on the electronic behaviour of the bulk graphite. “Such an effect would be imperceptible in typical metals since there are far more conducting charges in the bulk that would mask the properties of the moiré surface,” Yankowitz adds.
At large magnetic fields, an even more profound and exciting transformation takes place. Under these conditions, the electrons in the graphite form a standing wave that extends across all layers of the bulk material, effectively coupling the graphite-graphene surfaces together. The result, Yankowitz tells Physics World, is a “mixed-dimensional moiré material” in which “properties of the single rotated 2D interface effectively become hybridized with the 3D bulk”.
Flat Landau bands
To understand the physics behind these observations, Yankowitz and colleagues drew on previous work by researchers at Manchester. “We were fortunate that [the Manchester team] had recently worked out the physics behind the unusual standing-wave properties of (untwisted) graphite in a large magnetic field,” he explains. “This work turned out be the key to eventually understanding the complex physics in our 2D-3D moiré hybrid structure.”
A single layer of graphene can be described as a simple repetition of carbon atoms arranged in a crystal structure known as a unit cell. In a moiré superlattice of two graphene layers stacked on top of each other with a small twist angle between them, this unit cell expands to a huge extent, as if the 2D crystal was artificially stretched a hundred times. This stretching causes the material’s electronic band structure to become flattened, dramatically changing its interactions and properties.
In the specific case of the 2D-3D moiré hybrid, Yankowitz says that the coupling across graphene and graphite layers stems from the formation of so-called flat Landau bands. These flat bands only occur in a handful of physical systems, and they give rise to unusual quasi-1D electronic states that explain the phenomena he and his colleagues observed.

A 2.5D Hofstadter’s butterfly
Independently, a Manchester team led by Artem Mischenko has also been exploring the effects of moiré potentials on bulk graphite. After calculating that such effects could penetrate more than 40 layers of graphitic atoms, Mischenko and colleagues performed experiments on a different 2D-3D hybrid: bulk hexagonal graphite crystallographically (mis)aligned with hexagonal boron nitride, a 2D material sometimes called “graphene’s cousin”.
In this system, the Manchester researchers observed a 2.5-dimensional mixing of the surface and bulk states in graphite. This mixing manifests itself in a 2.5D version of Hofstadter’s butterfly, which is a striking fractal pattern that arises in the energy levels of electrons moving in a magnetic field while confined in a lattice. The effect can also be understood as a new type of fractal fractional quantum Hall effect.
According to Vladimir Fal’ko, a theoretical physicist at Manchester and director of the UK National Graphene Institute, the new 2.5D quantum Hall effect in graphite stems from the interplay between two textbook phenomena in quantum physics: Landau quantization in strong magnetic fields and quantum confinement, “leading to yet another new type of quantum effect”.
Magic-angle graphene switches from superconductor to ferromagnet
While applications for these discoveries are not yet clear, Yankowitz says that both studies will advance our fundamental understanding of moiré physics. “We are now thinking about exploiting the 2D-3D hybridization effect we have observed to study more complex device geometries, such as multiple twisted interfaces within a single bulk graphite film to see if these hybridize in interesting new ways,” he says.
The two studies are described in back-to-back papers in Nature.