
Time crystals are special quantum systems that exhibit periodicity in time, just as crystalline materials are periodic in space. Since 2012, when they were first proposed theoretically, several groups have built experimental systems that demonstrate key characteristics of time crystals, but these results lacked a method to generally stabilize the time crystalline phase. An international team of researchers has now gone beyond previous experiments by creating a type of time crystal known as a discrete time crystal (DTC) out of a chain of programmable spin quantum bits (qubits). The new system exhibits a phenomenon known as many-body localization that prevents the DTC from heating up and thermalizing and is considered a “smoking gun” that demonstrates its status as a genuine time crystal.
While time crystals are generally analogous to spatial crystals in terms of exhibiting periodicity across a dimension, the time periodicity of a DTC is subtly different. Specifically, a DTC’s behaviour repeats itself over an integer multiple (hence the name “discrete”) of the period of the force that drives it to change its quantum state.
To qualify as a time crystal, the system must meet two criteria: it must be robust to fluctuations (similar to normal crystals), and the energy that drives it must not cause it to heat up. This second requirement imposes special conditions on the system, because whereas most physical systems absorb energy to reach thermal equilibrium, for a time crystal such heating leads to the system’s destruction.
The way to avoid this is known as many-body localization (MBL), which essentially means creating a many-body system in which a certain amount of disorder is introduced. Although the particles inside such a system couple with different states, they do so with random variations, leading to destructive interference. The disorder therefore prevents the system from heating up, maintaining its stability.
A new spin
In the latest study, which is published in Science, researchers at QuTech (a collaboration between TU Delft and TNO in the Netherlands), the University of California, Berkeley, and the industrial diamonds firm Element Six created a DTC from nuclear spins in carbon-13 atoms located near a defect in diamond’s crystal lattice. These defects are known as nitrogen-vacancy (NV) centres, and their electronic spin can be controlled using optical techniques. This capability allows the nuclear spins in nearby carbon-13 atoms to be addressed as well, meaning that the nuclear spins act as well-isolated qubits with quantum states that are both controllable and detectable. To meet the criteria for MBL, the system should contain disorder. The diamond spin system achieves this because the position of the carbon-13 nuclear spins within the lattice is not ordered, which naturally causes variations in the coupling between spins.
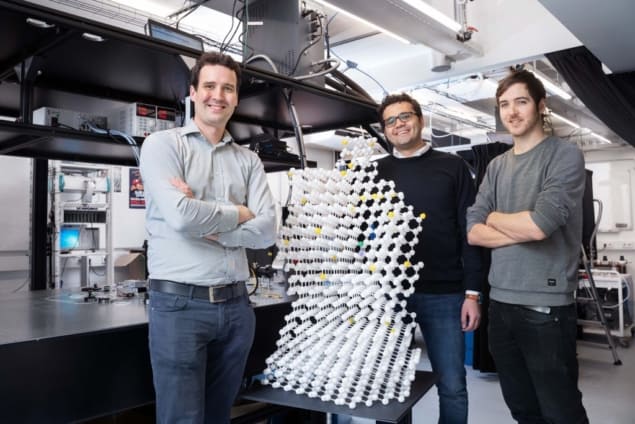
To create the system’s initial state, the researchers set the all the nuclear spin polarizations to be the same. They then selectively altered these states by applying radiofrequency (RF) pulses. After one cycle, they retrieved the system’s initial state, meaning the cycle could start again. The period of this cycle was twice the period of the driving RF pulses – a characteristic of DTC behaviour – and the researchers found they could maintain the pattern over 800 cycles, for a total of eight seconds. The key DTC signature, however, came from altering the initial state of the many-body system and repeating the cycle. Regardless of system’s initial state, the experiment formed a stable DTC. This robustness was the key element the team was looking for to show that the time crystal was being stabilized by disorder in its internal interactions and not by prethermal effects that exponentially slow down the heating.
Perfect timing
Tim Taminiau, the QuTech physicist who led the research, says that the stability of time crystals is connected to fundamental questions in non-equilibrium statistical mechanics. “This work provides experimental input to such puzzles and helps lay the groundwork for understanding the scenarios in which time crystals appear and what their observable signatures are,” he says. He notes that another collaboration has reported a similar result on arXiv, using a quantum processor based on superconducting qubits. “It is exciting to see two experimental breakthroughs happen so shortly after another,” he says. “Both results are complementary: they used two times more qubits, but our time crystals lived ten times longer.”
As for future work, Taminiau points out that “a perfectly isolated time crystal can, in principle, live forever, so extending the lifetime is our next step”. To investigate the various settings in which time crystalline behaviour occurs, he and his colleagues want to study what happens in 3D systems (rather than the 1D case discussed here), as well as in other driven phases of matter such as topological phases. From a broader perspective, he adds that the group now has a powerful new quantum simulator with individual control over all the qubits, which can be used to investigate “a large variety of interesting problems in many-body physics and other fields”.
Time crystals enter the real world of condensed matter
Peter Hannaford, a physicist at Swinburne University of Technology in Melbourne, Australia, who was not involved in the research, describes the result as being important for the field of time crystals, as the QuTech-led team unambiguously demonstrated a many-body localization DTC for the first time. He suggests that previously reported experimental results on DTCs based on spin systems were likely to be stabilized by prethermal effects, or else they did not employ disorder. As for future work, he notes that this study and the similar result currently on the arXiv both have two temporal lattice sites. “An exciting challenge for the future is to explore bigger DTCs with 100 or more temporal lattice sites,” he says. “Those can be ideal for studying (exotic) condensed matter phenomena in the time dimension and might be realized, for example, from a Bose-Einstein condensate of ultracold atoms bouncing resonantly on an oscillating mirror.”