Canadian technology start-up Medscint is partnering with researchers at UMC Utrecht to develop a real-time dosimetry platform for MR-guided adaptive radiotherapy
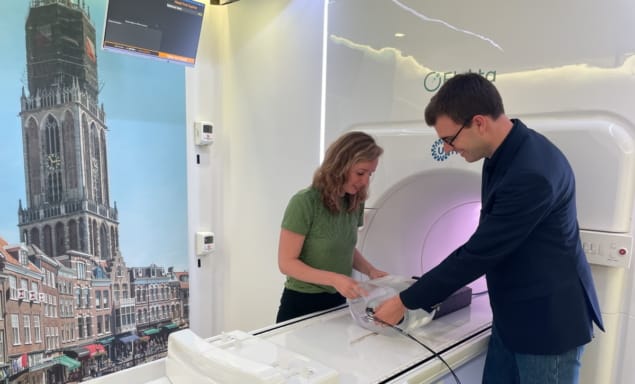
Tumour shape and position relative to healthy tissue will evolve as a cancer patient undergoes a course of radiotherapy – and can even change during an individual treatment session. The ability of MR-guided radiotherapy (MR/RT) systems like the Elekta Unity MR-Linac to detect those changes and adapt therapy accordingly – in effect, helping clinicians to “see what they treat” in real-time – represents a fundamental inflection point for the radiation oncology team.
While it’s still relatively early days for MR/RT adoption, the clinical end-game is already coming into view. Think personalized radiotherapy tailored to the unique indications of each patient – adjusting radiation delivery to address the daily variation in the tumour and surrounding healthy tissue, while enabling the clinician to adapt the plan for tumours that respond rapidly to treatment, as well as those that prove unresponsive to standard doses of radiation. Ultimately, researchers hope that the MR-Linac’s ability to visualize a tumour target with exceptional soft-tissue contrast, both prior to and during treatment, will make it possible to increase the radiation dose to diseased tissue in real-time without damaging adjacent organs-at-risk (OARs) and other critical structures.
Real-time adaptation
In the vanguard of this adaptive MR/RT research effort are Martin Fast and colleagues at UMC Utrecht in the Netherlands. For context, UMC Utrecht was the first research hospital to “go clinical” with high-field MR/RT – back in 2017 – and the centre’s radiation oncology department now has three Elekta Unity systems in routine daily operation. “We’re currently doing adaptation just before treatment starts and sometimes midway through a course of radiotherapy,” explains Fast, associate professor of medical physics at UMC Utrecht. “Where we’re heading, though, is continuous online adaptation of radiation delivery – a breakthrough that will allow us to take account of changes in tumour shape and position as a result of respiratory and cardiac motion.”
Fundamental to success here is a convergence of core enabling technologies. For starters, there’s the ability to capture the tumour and its environment “on the fly” using the MR-Linac’s real-time imaging capability. Put simply, novel MR imaging sequences are able to map a wide range of tumour motion – when the patient breathes, for example, or as a result of peristaltic activity along the digestive tract – which opens the way to dynamic tracking of the linac’s multileaf collimator (MLC), continuously reshaping the treatment beam so as to confine dose to the tumour target while avoiding OARs and surrounding healthy tissue.
Reliable, end-to-end QA is equally fundamental for the successful translation of breakthrough technologies into the radiotherapy clinic. A key building block in the MR/RT QA workflow at UMC Utrecht is the QUASAR MRI4D, an MR-safe, programmable motion phantom from Modus QA, a Canadian supplier of QA solutions for radiation oncology. As well as being a preferred QA vendor, Modus has a long-standing R&D partnership with the UMC Utrecht team – a dialogue that’s proved instrumental in addressing another piece of the MR/RT QA puzzle. “We were brainstorming new ways of measuring dose dynamically,” adds Fast, “and Modus introduced us to Medscint and their real-time, small-field dosimetry solution based on plastic scintillation detectors.”
Making light work of MR/RT QA
Based in Québec City, Medscint is a young and growing technology company combining expertise in photonics, scintillation dosimetry and medical physics. In terms of specifics, the start-up’s plastic scintillators combine near-water-equivalence and real-time response with high spatial resolution and MR-Linac compatibility. The scintillation detectors – known commercially as the HYPERSCINT Research Platform – also offer multipoint capability with a compact footprint (0.5 mm long, 0.5 mm diameter), which makes them ideal for small-field dosimetry and novel phantom developments.
After exploratory conversations at the end of last year, the UMC Utrecht/Medscint collaboration has proceeded at pace. The partners jointly presented on the characterization of the scintillation detectors in an MR-Linac environment at the Canadian Organization of Medical Physics (COMP) Annual Scientific Meeting last month. Meanwhile, new results demonstrating multipoint time-resolved plastic scintillation dosimetry on an MR-linac will feature at the AAPM Annual Meeting in Washington DC next week. This latest work is part of a collective effort – also involving scientists and engineers from Elekta and Modus – to road-test an experimental set-up in which a prototype 3D-printed hybrid cassette (containing four scintillation detectors and one EBT3 film) is incorporated in the QUASAR phantom to quantify MLC tracking for lung stereotactic body radiotherapy.
“We want to use a dosimeter that will integrate easily with the QUASAR motion platform,” explains Prescilla Uijtewaal, a PhD student at UMC Utrecht who co-led the design and validation work on the hybrid cassette. What’s more, the plastic scintillation dosimeter allows the end-user to see how dose is developing in real-time without being influenced by MR image acquisition, the scanner’s magnetic field or the motion of the phantom. Unlike other detectors, the HYPERSCINT platform can also be used regardless of its orientation in a magnetic field environment. “In that sense,” adds Uijtewaal, “it’s a pretty straightforward dosimetry device, because we don’t have to worry about all these dependencies.”
Plastic scintillation detectors ready to shine as FLASH radiotherapy gathers momentum
Fast, Uijtewaal and colleagues are already in talks to extend the strategic partnership with Medscint, with plans to evaluate more real-time dosimetry use-cases in the MR-Linac environment. One work-in-progress initiative involves the use of deformable inserts for the QUASAR phantom, mirroring the complexity and 4D deformation that tumours are subjected to as a result of motion within the body – and notably so for radiotherapy treatments in the cardiac region.
“The heart’s motion is not just rigidly translating – it’s deforming with each heartbeat,” explains Fast. “As such, we plan to integrate the HYPERSCINT detectors with a realistic model that supports time-resolved dosimetry while taking account of this type of complex motion and deformation.”
Big opportunities in small-field dosimetry
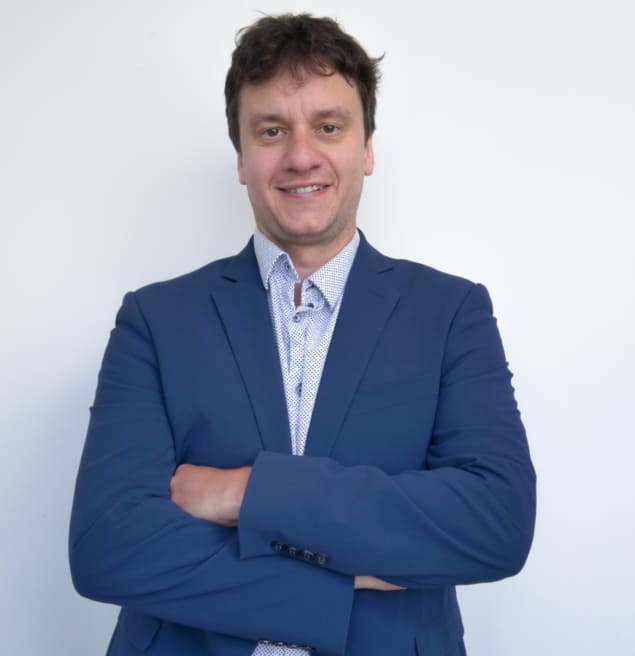
François Therriault-Proulx, president and CEO of Medscint, was one of the company’s co-founders in 2016, along with colleagues Simon Lambert-Girard (chief science and technology officer) and Jonathan Turcotte (chief product, sales and marketing officer). Prior to establishing Medscint, Therriault-Proulx spent eight years as an academic scientist working on the fundamentals of scintillation dosimetry, spanning PhD and postdoctoral research positions at Université Laval, Quebec City, and the University of Texas MD Anderson Cancer Center, Houston. He talked to Physics World after his presentation at last month’s AAPM Summer School on Small-Field Dosimetry, SRS and SBRT.
How did delegates respond to your talk at the AAPM summer school?
There were around 180 attendees at the summer school – mostly medical physicists. For many delegates, my presentation offered the first opportunity to learn about plastic scintillation detectors in terms of the fundamental science and enabling technologies under development. As such, the event was an ideal forum for Medscint to get the word out to the clinical physics community, raising awareness about the HYPERSCINT Research Platform, the capabilities of our in-house product development team, also our R&D partnerships on cutting-edge applications like adaptive MR/RT and FLASH radiotherapy.
Why are scintillation detectors a good fit for small-field dosimetry?
Compact-footprint, water-equivalent plastic scintillator technology has a lot to offer as treatment fields get smaller and geometrically more complex – for example, in SRS treatments of metastatic tumours in the brain. With no need for small-field correction factors to characterize device behaviour, our detectors provide a real-time measurement tool that combines high linearity with respect to dose and dose rate. That wide linear dynamic range is relevant at both ends of the treatment spectrum, whether for novel low-dose-rate irradiation schemes or ultrahigh-dose-rate FLASH applications.
What are the priorities for Medscint at the AAPM Annual Meeting this month?
First up, we’re looking to establish new collaborations with radiotherapy research groups, though our direction of travel is shifting towards cross-disciplinary teams with a focus on clinical translation. Second, we want to gather granular feedback from clinical end-users on their evolving QA requirements for next-generation radiotherapy modalities. We’ll also be exploring potential synergy with other technologies and partnership opportunities with relevant manufacturers.