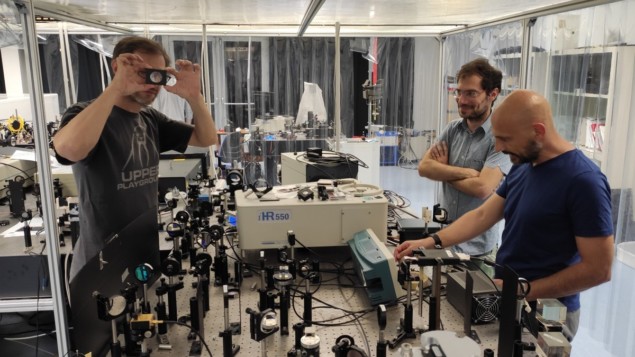
A bound state in the continuum (BIC) is a symmetry-protected topological state that, despite lying in the continuum radiation spectrum of a system, is unable to radiate in the far field. When supported by a photonic crystal, it is characterized by a supposedly infinite lifetime and a remarkable ability to enhance non-linear effects.
Now, researchers in Italy, the US and France have utilized these properties to demonstrate polariton Bose-Einstein condensation in a planar waveguide in which semiconductor quantum wells are strongly coupled to a BIC. The particularly low excitation threshold of the system shows promise for creating easily-integrable hybrid light-matter optical circuits and quantum information processing systems. The full study is described in Nature.
Hybridization of light and matter
Due to their bosonic nature, exciton-polaritons (quasiparticles made from photons coupled to electron-hole pairs) can undergo a Bose-Einstein-like condensation, in which a macroscopic number of polaritons condense into the lowest energy quantum state. Light emitted from such systems mimics laser emission and polariton condensates are therefore sometimes called “polariton lasers”. These systems also have important applications in quantum computing and all-optical devices.
Extremely high particle densities are required to create such condensates, so they are generally realized in high-quality optical microcavities with long photon confinement times. However, these microcavities require complicated fabrication processes and cannot be easily integrated into electronic devices. As an alternative to microcavities, planar waveguides have recently been shown to support increased polariton confinement and easy tuning of polariton-polariton interactions, as well as simpler integration.
In this study, Vincenzo Ardizzone, a post-doctoral researcher at CNR-Nanotec in Lecce, and colleagues designed and built a planar waveguide that supported a BIC with zero intrinsic linewidth (i.e. an infinite lifetime). The waveguide was a layered structure consisting of 12 gallium arsenide quantum-well layers separated by aluminium gallium arsenide barriers, with a 1D grating etched into the top five layers. The grating lattice constant was chosen such that the BIC state was resonant with the excitonic transition of the quantum wells. This facilitated strong coupling between the light and matter, forming exciton-polaritons that, due to the presence of the BIC, are strongly localized within the grating and possess infinitely narrow linewidth.
Lowering the threshold
Using high energy laser pulses to excite the waveguide, the researchers then demonstrated polariton Bose-Einstein condensation. This was evidenced by the appearance of a double-peaked emission at the edges of the BIC in momentum space; a non-linear increase in emission intensity; a narrowing of the linewidth- and a blueshift — with the latter three effects being fingerprints of polariton condensation.
Ghost surface polaritons seen for the first time
The laser excitation threshold of 3 µJ/cm2 is significantly lower than previously reported for both photon and polariton lasers. Notably, it has previously been shown that polariton condensation was not possible in a similar structure that did not contain a BIC, demonstrating that it is necessary to facilitate a sufficient build-up of the polariton population.
Topological thinking
The researchers also demonstrated that the topological properties of the BIC were exhibited by the polaritons both below and above the condensation excitation threshold. By measuring the polarization of the emission, they demonstrated the existence of polarization vortices – which relate to a system’s topological charge – in both regimes. This is highly significant as it shows that it is possible to transfer the topological charge of a BIC to a Bose-Einstein condensate, providing an alternative platform for studies on topological systems.