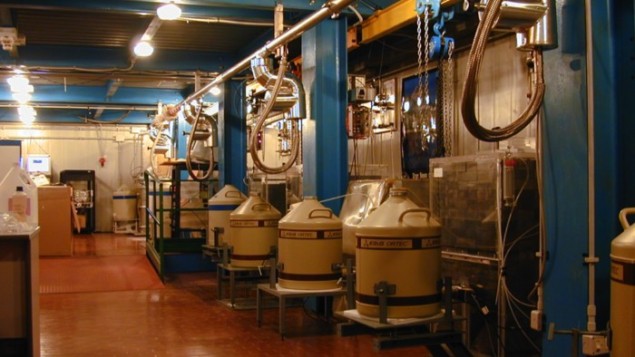
A controversial theory put forward by physicist Roger Penrose and anesthesiologist Stuart Hameroff that posits consciousness to be a fundamentally quantum-mechanical phenomenon has been challenged by research looking at the role of gravity in the collapse of quantum wavefunctions. Based on results from an experiment done under Gran Sasso mountain in Italy, the new work concludes that Penrose’s and Hameroff’s Orchestrated Objective Reduction theory (Orch OR) is “highly implausible” when based on the simplest type of gravity-related wavefunction collapse – although they point out that more complex collapse models leave some wiggle room.
Many scientists regard consciousness as a global manifestation of individual calculations by the brain’s billions of neurons. Penrose and Hameroff instead argue that consciousness is based on the non-computational collapse of coherent quantum superpositions between cellular structures within neurons known as microtubules. They reckon that while the superpositions guide classical neuronal processes, it is the continual gravity-related collapse of the quantum states that gives rise to our sense of self-awareness.
In the latest work, Catalina Curceanu of the Frascati National Laboratory near Rome and colleagues assess the plausibility of Orch OR in the light of results from an experiment they set up to probe gravity’s possible role in wavefunction collapse. Standard quantum theory leaves open the question of what causes a state’s wavefunction to collapse, simply providing the probabilities of the system collapsing into one classical state or another and implying that the process is random. But several physicists over the years have attempted to identify a physical mechanism behind the process – among them Penrose and Lajos Diósi, who have developed the Diósi–Penrose model. Diósi is at Hungary’s Eötvös Loránd University and Wigner Research Centre for Physics and has worked with Curceanu on this latest research.
Different curved spacetimes
The Diósi–Penrose model involves combining quantum mechanics with classical gravity such that a spatial superposition of quantum states generates a superposition of different curved spacetimes. The idea is that the latter superposition is unstable and causes the system’s wavefunction to collapse when the gravitational energy resulting from the difference in spacetime formations – and therefore system mass – exceeds some threshold. This process is independent of wavefunction decoherence by environmental noise, but its realization requires that the latter is kept at bay.
While both Penrose and Diósi arrived at the same simple formula for the timescale over which this type of collapse would occur, their individual models differ. Penrose did not specify the dynamics of wavefunction collapse, whereas Diósi provided a full dynamical description. In doing so Diósi predicted that collapse should be accompanied by the emission of electromagnetic radiation – generated by charged particles within the system as they undergo a continuous Brownian motion related to the collapse mechanism.
Now, Diósi teamed up with Curceanu and other physicists in Italy and Germany to establish whether his predicted radiation really is given off in nature. The group did so by monitoring the emissions from a cylinder of germanium about the size of a small tin of beans shielded from external radiation by lead and copper shields as well as the 1400 m of rock above the lab – the Gran Sasso National Laboratory near L’Aquila. They were able to test the Diósi–Penrose model by working out how much gravity-related collapse radiation should have been produced by the charged particles within the germanium and comparing their calculations against the measurements.
Carrying out their experiment over the course of two months in the summers of 2014 and 2015, they measured no radiation beyond that expected from residual emissions in the experimental apparatus. This allowed them to impose a lower limit on a parameter, R0, that they describe as the effective size of the particle’s mass density.
That result already ruled out one specific and natural formulation of the Diósi-Penrose model – which stipulated that the scale of the superposition is comparable to the size of the nuclei themselves – the measured lower limit of R0 being 0.54×10-10 m while the size of the nuclear wavefunction of germanium cooled to liquid-nitrogen temperatures (as was the case in their experiment) would be an order of magnitude lower (0.05×10−10 m).
Molecular scale
Now, Curceanu, Diósi and colleagues have analysed what that value of R0 means specifically for the Orch OR theory, assuming two distinct scales of superposition – the nuclear one favoured by Penrose (about 10-15 m) and one similar to the size of whole tubulin proteins within a strand of microtubule (about 3 nm). In each case their aim was to work out how much brain matter would be needed to collapse the wavefunction on a timescale comparable to that of conscious experiences (typically about 0.5 s, but potentially as brief as 0.025 s).
Do quantum effects play a role in consciousness?
With a nuclear-sized superposition, the collapsing effect of individual carbon nuclei within tubulin proteins is miniscule and therefore calls for huge numbers of nuclei to act in concert. In fact, the researchers work out that to collapse the wavefunction in around 0.025 s a whopping 1023 tubulins would need to make up the coherent state. But as they point out, there are reckoned to be only 1020 tubulins in the whole brain (about 109 in each neuron). “These considerations seem to rule out tubulin separation at the level of the atomic nuclei,” they say.
In the second scenario, the larger superposition scale implies that fewer tubulins would need to remain coherent. Indeed, Curceanu and colleagues work out that a mere 1012 would do the job. Still, they say, the overall requirements seem daunting – the brain needing to maintain a mass of 10−16 kg in a coherent state for 25 ms over a length scale of about 10 nm. “This vastly exceeds any of the coherent superposition states achieved with state-of-the-art optomechanics or macromolecular interference experiments,” they note.
The researchers add that not all is lost for Orch OR. While they reckon that the theory seems implausible if based on the simplest wavefunction collapse model, it may become more plausible if a more sophisticated model can be developed – one, for example, that conserves energy (something not true of Diósi’s current model). “In future work,” they say, “we intend to develop such variants of the Diósi-Penrose collapse dynamics and then reexamine the tubulin superposition scenarios discussed above”.
The research is described in Physics of Life Reviews.