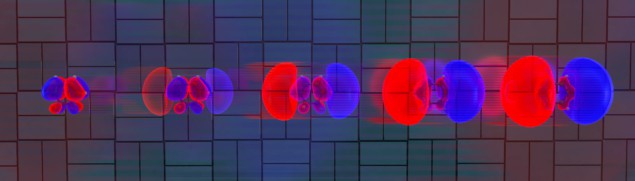
Scientists in the US and UK are the first to observe electrons rearrange their positions in molecules during the early stages of a light-driven chemical reaction. They did so by firing ultrashort light and X-ray pulses at the molecules to create “movies” of electron motion. The technique promises to shed further light on chemical processes such as bond making and breaking. Their method could also be used to study other ultrafast processes in physics, chemistry, and biology.
There are many chemical reactions that are driven by light. Some, such as photosynthesis in plants and vitamin D production in skin are desirable, while others such as the fading of paint in sunlight are not. Chemists have long predicted that the first step in light-driven reactions is the rapid rearrangement of electrons, while the atomic nuclei move much more slowly.
“Once the electrons have changed their positions, the forces acting on the nuclei will change, leading to atomic motion and further physical processes such as energy or charge transport,” explains Adam Kirrander at the University of Edinburgh. Now, Kirrander along with colleagues at Edinburgh, Brown University and the SLAC National Accelerator Laboratory have used X-ray scattering to track this rearrangement in real time.
Electron distribution
X-ray scattering is a popular technique for determining the positions of atoms within crystals and molecules. But the X-rays interact mostly with the atomic electrons – rather than the atomic nuclei – so X-ray scattering actually reveals the distribution of electrons within a material or molecule. This distinction does not usually matter for a sample at equilibrium, but during a chemical reaction the fast-moving electrons will begin rearranging themselves well before the lethargic and much heavier atoms start to move. As a result, ultrashort X-ray pulses can be used to observe this electronic motion.
Doing so involves firing an ultrashort light pulse at sample of molecules – which sets the reaction in motion. This is followed almost immediately by an ultrashort X-ray pulse, which takes a snapshot of the electron distribution of the molecules as the reaction progresses. By varying the delay between the light and X-ray pulses, a movie of the changing distribution of electrons can be made.
To see this motion, the lengths of the two pulses and the delay between must be on the order of tens of femtoseconds. However, is not possible to have complete control of the delay between the light and X-ray pulses. “We can get the time delay between the pulses roughly right,” explains Kirrander. “But after this we rely on something called “timestamping”, which is a diagnostic tool that records the exact time delay between the optical and the X-ray pulse”.
Five years ago, the team performed such an experiment using pulses from the X-ray Free-Electron Laser at the Linac Coherent Light Source at SLAC. They were able to follow the movement of atoms in 1,3-cyclohexadiene molecules as a function of time, but the time resolution of the experiment was not good enough to see the electrons move independently of the atoms.
Interesting molecule
The species 1,3-cyclohexadiene was used because it undergoes a large change in electronic structure and is therefore favourable for making X-ray movies. However, Kirrander points out that it is an “interesting molecule that serves as an important model for more complex biological reactions like the one that produces vitamin D when sunlight hits your skin”.
Coming soon: Rydberg the movie
Now, the team has improved the experiment and data-analysis techniques and are now able to watch the preliminary motion of the electrons as light pulses hit 1,3-cyclohexadiene. They found that the electron distribution ballooned in size over a period of 30 fs (see figure).
SLAC senior staff scientist Michael Minitti explains, “We’re imaging these electrons as they move and shift around. This paves the way to watching electron motions in and around bond breaking and bond formation directly and in real time”.
Kirrander points out that the technique could be used to study a range of systems – however it could be tricky to apply it to situations where the electrons and atoms move on the same timescale. “With further improvements in the experiments and the data analysis, we anticipate that we will be able to simultaneously capture changes in electronic structure and atomic positions throughout complex dynamic processes.”
The research is described in Nature Communications.